Crystallogenesis
Crystallization of the target bio molecules is a requirement to perform structural analysis by X-ray crystallography. However, crystallization of biomolecules is sometimes difficult to achieve. Therefore, one main research topic of the group, together with industrial partners, is the development of innovative and advanced technologies and equipment for analysis, directed optimization and scoring of crystallization experiments. One goal of this research and instrumentation activities is to develop methods to predict at an early stage the crystallization process, if suitable crystals will grow or not; other research activities along this topics are briefly summarized. Projects are supported via EU, BMBF, DFG or DLR grants.
The Xtal Controller- Feedback controlled Navigation through the Phase Diagram
Growth and preparation of high quality micro- sized crystals optimal for data collection experiments at modern micro-beam insertion-device synchrotron (SR) beamlines and growth of nano- crystals required for data collection at Free-Electron-Laser (FEL) beamlines is a challenging task. Therefore new and advanced methods to prepare and score 3D micro- and nano- crystal suspensions, most suitable for X-ray and electron diffraction experiments are in focus of research activities within the group. For a successful crystallization, the knowledge and control of solutions concentrations and associated time dependent processes is of fundamental importance. In terms of industrial collaboration we developed a unique hardware system, the XtalController, which allows a controlled navigation in the phase diagram of protein crystallization. The initial principles of the XtralController were established in terms of PhD thesis and today we are approaching the 4th hardware generation. Data obtained from the XtalController provide new information and more insights about the early processes during the nucleation and self-assembly process of liquid dense clusters and nanocrystals (Ref. and Patent). The growth kinetics of these protein clusters, forming distinct radii fractions, is analyzed in real-time by Dynamic Light Scattering (DLS). Latest data provide experimental evidence that during nucleation, a formation of distinct liquid clusters with high protein concentration occur prior to a transition to crystal nuclei by increasing the internal structural order of these clusters, subsequently.
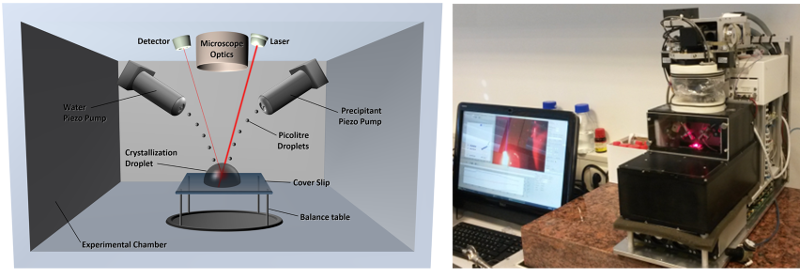
Schematic (left) and photographic (right) presentation of the temperature- and humidity-controlled experimental chamber of the XtalController instrument.
In vivo Crystallization
The advent of the X-ray free-electron laser (XFEL) radiation and the establishment of serial crystallography (SFX) opened new avenues in structural analysis. The successful structure elucidation of cathepsin B applying micro-crystals we prepared first time in already in 2007 in collaboration with Prof. Duszenko (Tübingen), obtained by in vivocrystallization in baculovirus infected Sf9 insect cells, clearly proved that crystals grown intracellular are very well suited for XFEL sources. We continued to analyse and optimize the process of in vivocrystallization and could proof the high potential of preparing quantities required for SFX data collection at novel highly brilliant XFEL as well as at synchrotron radiation sources. In principle it is very well known that protein crystals may appear under in vivoconditions in living cells, like peroxidase in peroxisomes, insulin in secretory vesicles of pancreatic cells, storage proteins in plant seed and others (Doye & Poon, 2006). These proteins are produced in large quantities and usually appear within membrane surrounded organelles that offer only limited space and volumes. And formation of protein crystals seems the simplest way to produce the highest possible number and concentration of individual protein molecules and they may appear as a result of the displacement of surrounding water due to space restrictions within organelles. Although the detailed molecular and cellular principles of protein in vivo crystal growth is till now not elucidated so far, the cell could provide suitable environmental conditions for crystal nucleation within a specific compartment, comparable to crystal formation obtained applying in vitro methods and crystallization compartments in the lab. We apply complementary bioanalytical and biophysical methods to obtain more insights about crystallization in cells and established a pipeline for systematic analysis and production of in vivo grown cyrstals.
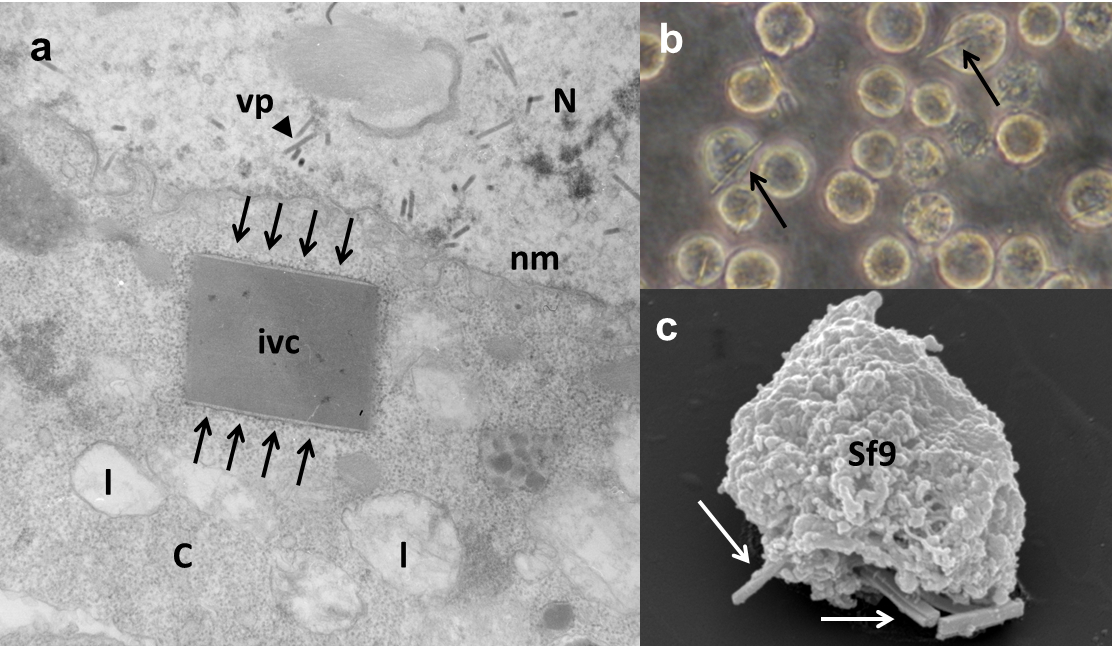
In vivo crystals inside Sf9 cells. (a) Electron micrograph showing an in vivo crystal, surrounded by a membrane, within the cytoplasm. (b) Light microscopic image showing Sf9 insect cells containing in vivo crystals. (c) Scanning electron micrograph image of in vivo crystals protruding from Sf9 insect cells.
Liquid Dense Clusters
As the analysis of structure and dynamics of macromolecules and their complexes, without necessarily being constrained to crystalline samples, is coming in focus at XFEL radiation sources, the rather newly-identified phase of macromolecular solutions, called protein dense liquid clusters (PDLCs) are of interest to be applied as samples or sample suspensions. These clusters have already been found to show certain local ordering of the constituents and they therefore can be considered to some extend as intermediates between nanocrystals and single particles. These structures have also only very recently been recognized to be associated with organelles, tubules, and other cellular structures. In this context PDLCs have the potential to be used for future single particle diffraction and imaging experiments at the European XFEL. In terms of research activities also with partners at DESY and CFEL we analyse the chemical and physical behavior of such clusters and their potential to be utilized for future time resolved imaging approaches at synchrotron-radiation facilities and X-ray FELs. PDLCs serve also as a basis to study dynamic order/disorder phenomena, which can be influenced or triggered by chemical or physical effects and may also support investigations of large structural motions and fluctuations introduced for instant by receptor-ligand, or membrane-protein interactions. These large structural changes cannot be tolerated by a rigid crystal lattice and therefore cannot be assessed by crystallography. Utilizing PDLCs could therefore open new ways in structural biology and drug discovery, following time resolved ligand binding, as well as providing new insights in protein dynamics at high spatial and time resolution.
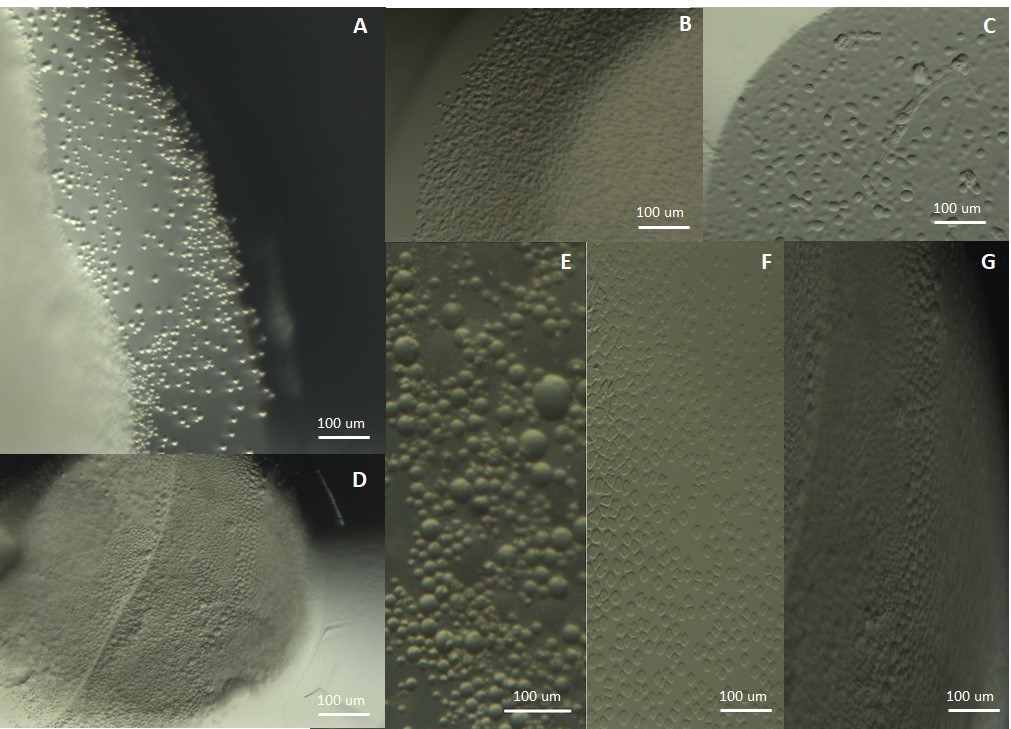
Clusters obtained: (A) Trypsin, (B) BSA, (C) Egg albumin, (D) BSA, (E) tRNA, (F) Glucose Isomerase and (G) BSA.
Depolarized Dynamic Light Scattering for Nanocrystal Detection
Methods for preparation and scoring of protein nanocrystal suspensions, to be used for serial femtosecond crystallography (SFX) experiments, are developed and optimized within the group. The identification of the presence of a spatial repetitive orientation of macromolecules (crystal nuclei) in the early stages of the crystallization process is essential to detect nano-crystals. The optical properties of a crystal lattice offer the potential to detect the transition from disordered to higher ordered particles.
A unique experimental setup was designed and constructed in terms of a project funded by BMBF to detect nano-crystal formation by analyzing depolarized scattered laser light. The ability of a lattice to depolarize laser light depends on the different refractive indices along different crystal axes. The novel, non-invasive method is based on quantitative analysis of photon counts obtained from depolarized and polarized dynamic laser light scattering. The detection of nanocrystals exploits the potential of crystalline material to depolarize laser light owing to optical properties like birefringence. The efficient, fast and none invasive characterization of crystallization experiments in real time is today of fundamental importance for successful preparation of protein nanocrystals and nanocrystal suspensions to be applied for SFX diffraction data collection. All data, results and applied procedures, together with a description of the hardware, have been published in the Journal of Applied Crystallography (2015) 48: 1476-1484.
A unique experimental setup was designed and constructed in terms of a project funded by BMBF to detect nano-crystal formation by analyzing depolarized scattered laser light. The ability of a lattice to depolarize laser light depends on the different refractive indices along different crystal axes. The novel, non-invasive method is based on quantitative analysis of photon counts obtained from depolarized and polarized dynamic laser light scattering. The detection of nanocrystals exploits the potential of crystalline material to depolarize laser light owing to optical properties like birefringence. The efficient, fast and none invasive characterization of crystallization experiments in real time is today of fundamental importance for successful preparation of protein nanocrystals and nanocrystal suspensions to be applied for SFX diffraction data collection. All data, results and applied procedures, together with a description of the hardware, have been published in the Journal of Applied Crystallography (2015) 48: 1476-1484.
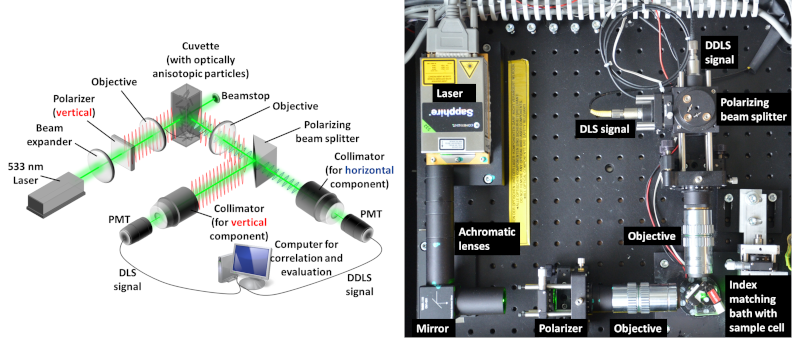
Scheme (left) and picture (right) of the Depolarized Dynamic Light Scattering instrument for nano-crystal detection in real-time.
Multi-channel Dynamic Light Scattering
Dynamic light scattering (DLS) is a most powerful, highly adaptable and non-invasive method to analyze the size distribution of various kinds of particles in solution in a time-resolved manner and in a rather wide size range. The intensity fluctuations of laser light scattered by particles in solution can be autocorrelated after short time-intervals in order to determine the respective diffusion coefficient. Samples can be studied in a variety of sample containers, e.g. in order to monitor the crystallization or assembly of macromolecules, which complements data obtained by small-angle X-ray scattering and most common spectroscopic techniques. Our group established a multi-channel detection principle to record scattering data in different neighboring volumes of a sample solution at the same time at the Small Angle X-ray Scattering (SAXS) EMBL beamline P12 at PETRA III (DESY), funded by BMBF. This setup allows obtaining additional sample information during SAXS data collection and thereby supports the evaluation of the obtained data. In addition an 8- channel DLS system suitable to investigate the mass transport phenomena in macromolecular crystallization process (funded by DLR) is currently in development as a benchtop instrument, see figures below.
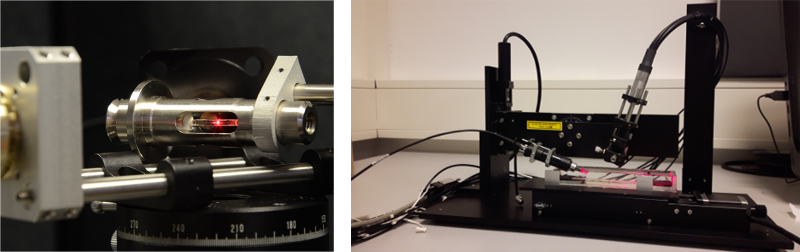
Multi-channel DLS system to investigate mass transport phenomena in macromolecular crystallization process.
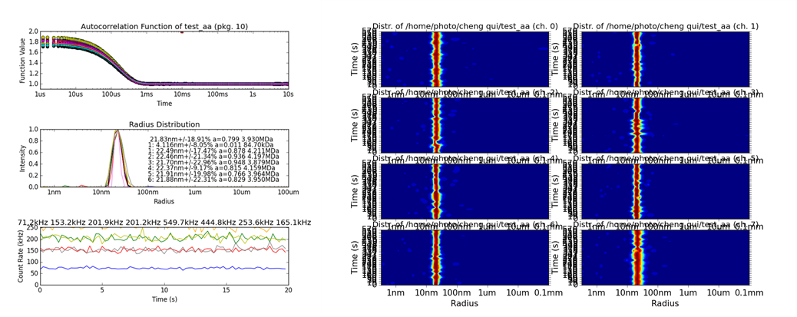
Laser light scattered by a sample solution inside a capillary. The setup allows varying the scattering angle of the DLS experiment.
Mass Transport in Crystallization in Microgravity Environment
Proteins crystallized in microgravity are of higher quality than those crystallized on Earth. Growing high quality protein crystals enhance the opportunity to obtain high resolution diffraction data, thus also enabling three-dimensional structure exploration for biological macromolecules more accurately. Our group in cooperation with scientists from University of Alabama in Birmingham within the framework of the NASA-DLR project “LMM Biophysics 1” explores those phenomena by examining the mass transport of macromolecules within the protein crystallizations process in microgravity. For this purpose scientifically interesting proteins, particular from pathogen species, are selected. Samples are transported with spacecrafts to the International Space Station (ISS) and return back to earth after approx. 1 or 6 month. Microgravity crystallization experiments and crystals obtained under microgravity conditions are analyzed and evaluated comparative with ground grown crystals.
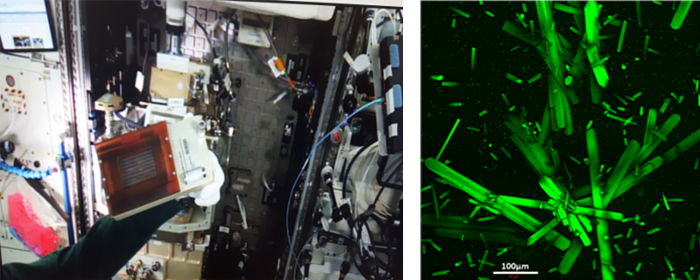
(Left): Flight cassette with samples from our group during installation to the LMM-Microscope on board of the International Space Station ISS (Feb.26, 2016) (right): Crystals in capillaries of a chemically modified protein, grown in microgravity condition on ISS.
Selected References
- S. Falke, H. Brognaro, C. Betzel: Latest Insights and Methods in Analysing Liquid Dense Clusters and Crystal Nucleation. Encyclopedia of Analytical Chemistry, 2018, in press.
- S. Falke.,K. Dierks, C. Blanchet, M. Graewert, F. Cipriani, R. Meijers, D. Svergun, C. Betzel (2018): Multi-channel in situ dynamic light scattering instrumentation enhancing biological small-angle X-ray scattering experiments at the PETRA III beamline P12. Journal of Synchrotron Radiation 25, 361-372 (2018).
- D. Baitan, R. Schubert, A. Meyer, K. Dierks, M. Perbandt, C. Betzel: Growing Protein Crystals with Distinct Dimensions Using Automated Crystallization Coupled with In Situ Dynamic Light Scattering. Journal of Visualized Experiments(2018), in press.
- R. Schubert, A. Meyer, D. Baitan, K. Dierks, M. Perbandt and C. Betzel: Real-Time Observation of Protein Dense Liquid Cluster Evolution during Nucleation in Protein Crystallization. Crystal Growth and Design 17, 954–958 (2017).
- R. Schubert, A. Meyer, K. Dierks, S. Kapis, R. Reimer, H. Einspahr, M. Perbandt and C. Betzel: Reliably distinguishing protein nanocrystals from amorphous precipitate by means of depolarized dynamic light scattering. Journal of Applied Crystallography 48, 1476–1484 (2015).
- M. Duszenko, L. Redecke, C. N. Mudogo, B. P. Sommer, S. Mogk, D. Oberthür, C. Betzel: In vivo protein crystallization in combination with highly brilliant radiation sources offers novel opportunities for the structural analysis of post-translationally modified eukaryotic proteins. Acta Crystallographica, Section F: Structural Biology Communications F71, 929-937 (2015)
- L. Redecke, K. Nass, D. P. DePonte, T. A. White, D. Rehders, A. Barty, F. Stellato, M. Liang, T. R. M. Barends, S. Boutet, G. J. Williams, M. Messerschmidt, M. M. Seibert, A. Aquila, D. Arnlund, S. Bajt, T. Barth, M. J. Bogan, C. Caleman, T.-C. Chao, R. B. Doak, H. Fleckenstein, M. Frank, R. Fromme, L. Galli, I. Grotjohann, M. S. Hunter, L. C. Johansson, S. Kassemeyer, G. Katona, R. A. Kirian, R. Koopmann, C. Kupitz, L. Lomb, A. V. Martin, S. Mogk, R. Neutze, R. L. Shoeman, J. Steinbrener, N. Timneanu, D. Wang, U. Weierstall, N. A. Zatsepin, J. C. H. Spence, P. Fromme, I. Schlichting, M. Duszenko, C. Betzel, H. N. Chapman: Natively Inhibited Trypanosoma brucei Cathepsin B Structure Determined by Using an X-ray Laser. Science 339, 227-230 (2013)
- R. Koopmann, K. Cupelli, L. Redecke, K. Nass, D. P. DePonte, T. A. White, F. Stellato, D. Rehders, M. Liang, J. Andreasson, A. Aquila, S. Bajt, M. Barthelmeß, A. Barty, M. J. Bogan, C. Bostedt, S. Boutet, J. D. Bozek, C. Caleman, N. Coppola, J. Davidsson, R. B. Doak, T. Ekeberg, S. W. Epp, B. Erk, H. Fleckenstein, L. Foucar, H. Graafsma, L. Gumprecht, J. Hajdu, C. Y. Hampton, A. Hartmann, R. Hartmann, G. Hauser, H. Hirsemann, P. Holl, M. S. Hunter, S. Kassemeyer, R. A. Kirian, L. Lomb, F. R. N. C. Maia, N. Kimmel, A. V. Martin, M. Messerschmidt, C. Reich, D. Rolles, B. Rudek, A. Rudenko, I. Schlichting, J. Schulz, M. M. Seibert, R. L. Shoeman, R. G. Sierra, H. Soltau, S. Stern, L. Strüder, N. Timneanu, J. Ullrich, X. Wang, G. Weidenspointner, U. Weierstall, G. J. Williams, C. B. Wunderer, P. Fromme, J. C. H. Spence, T. Stehle, H. N. Chapman, C. Betzel, M. Duszenko: In vivo protein crystallization opens new routes in structural biology. Nature Methods 9, 259-262 (2012)
- A. Meyer., K. Dierks, D. Hilterhaus, T. Klupsch, P. Mühlig., J. Kleesiek, C Betzel: Single-drop optimization of protein crystallization. Acta Crystallographica. Section F, Structural Biology and Crystallization Communications F68, 994–998 (2012).
- A. Meyer, K. Dierks, D. Hilterhaus, T. Klupsch, P. Mühlig, J. Kleesiek, R. Schöpflin, H. Einspahr, R. Hilgenfeld, C. Betzel: Single-drop optimization of protein crystallization. Acta Crystallogr. Sect. F Struct. Biol. Cryst. Commun. 68, 994-8 (2012).
- D. Oberthuer, E. Melero-García, K. Dierks, A. Meyer, C. Betzel, A. Garcia-Caballero, J.A. Gavira: Monitoring and scoring counter-diffusion protein crystallization experiments in capillaries by in situ dynamic light scattering. PLoS One 7(6):e33545 (2012).
- K. Dierks, A. Meyer, D. Oberthür, G. Rapp, H. Einspahr, C. Betzel: Efficient UV detection of protein crystals enabled by fluorescence excitation at wavelengths longer than 300 nm. Acta Crystallogr. Sect. F Struct. Biol. Cryst. Commun. 66, 478-84 (2010).
- K. Dierks, A. Meyer, H. Einspahr, Ch. Betzel: Dynamic light scattering in protein crystallization droplets: adaptations for analysis and optimization of crystallization processes. Cryst. Growth Des. 8, 1628-1634 (2008).